Modality Review: Introduction to Preclinical Optical Imaging
When to Consider Using Optical Imaging
When discussing Optical Imaging techniques in this series, the meaning is to cover bioluminescence (BLI) and fluorescence (FLI) imaging. This is a very specific use of the term optical, and although it can encompass a much larger area of imaging, the discussion here will be restricted to these two uses. In terms of preclinical imaging modalities, optical imaging offers a simplistic, yet highly sensitive, modality to perform molecular imaging.
The discovery of bioluminescent and fluorescent proteins has enabled researchers to produce cells that can be optically active, revolutionizing the capabilities of optical imaging for both bioluminescence and fluorescence applications. Optical probes are unique, in that they can be targeted to specific biomarkers or receptors, similar to radioactive tracers or MR-active agents, but fluorescent probes may be enzyme activated, or they may be produced by cells themselves, even under specific gene transcription factors. The same is true of bioluminescence enzymes which can be expressed by a wide variety of cells but can also be activated in specific environments due to gene transcription factors. In all cases, the development of fluorescent proteins and enzyme substrates that emit in the far-red spectrum has greatly increased the utility of these agents in vivo, due to the properties of light propagation through living tissue.
Bioluminescence imaging in small animals is quite a simplistic technique, requiring a suitable animal model with cells expressing luciferase, along with a dark room and CCD camera. This technique is highly sensitive, as there is no autofluorescence background, or interference from excitation wavelengths, resulting in relatively high-quality images. In this situation the sensitivity is so high that even very few cells can be tracked from deep inside the tissue.
There are several application areas where bioluminescence is most common, however none more than in tumor imaging. Tumor cells may be altered to express the luciferase enzyme. Once injected the presence of the living tumor cells can be detected with a high level of sensitivity. Models may be subcutaneous, but more likely be to orthotopic or metastatic tumor models.
Fluorescent imaging in small animals is also relatively simplistic, again a suitable animal model is required where either the fluorophore is expressed by specific cells within the animal, or an extrinsic agent is introduced. In this case, autofluorescence from tissues at specific wavelengths may be an issue, reducing the sensitivity of this technique. Excitation and emission wavelengths are also important when considering the depth of the signal within the imaging subject. Most fluorescent preclinical imaging systems utilize reflectance imaging, where both the light source for excitation and the detector are on the same side of the imaging subject.
Fluorescence imaging applications are more diverse, and may range from cell tracking, to biodistribution of a novel therapeutic compound. The possibilities are endless with transcription of genes under specific tissue microenvironments, such as hypoxia; or with fluorescence tags applied to antibodies or small molecules for the detection of specific biomarkers.
Multimodal Imaging
Optical imaging lends itself very well to multimodal imaging; that is that bioluminescence and/or fluorescence images can be acquired from the same subject sequentially, or a tomographic image may be co-registered with either a CT or MR image to provide anatomical context to the localized signal.
By combining the information gained through the highly sensitive optical imaging techniques with those providing anatomical context, one may be able to gather more information about a specific biological disease process, or the response to a new target compound.
As was discussed previously in this series, optical imaging may also be used as a screening tool, or precursor to ultrasound imaging – the approximate location of the signal may provide the ultrasound user with some indication of where to start looking for the tumor of interest. Combined optical and radionucleotide compounds may also be used to harness both the power of optical and PET/SPECT techniques.
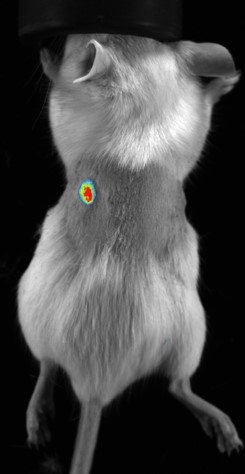
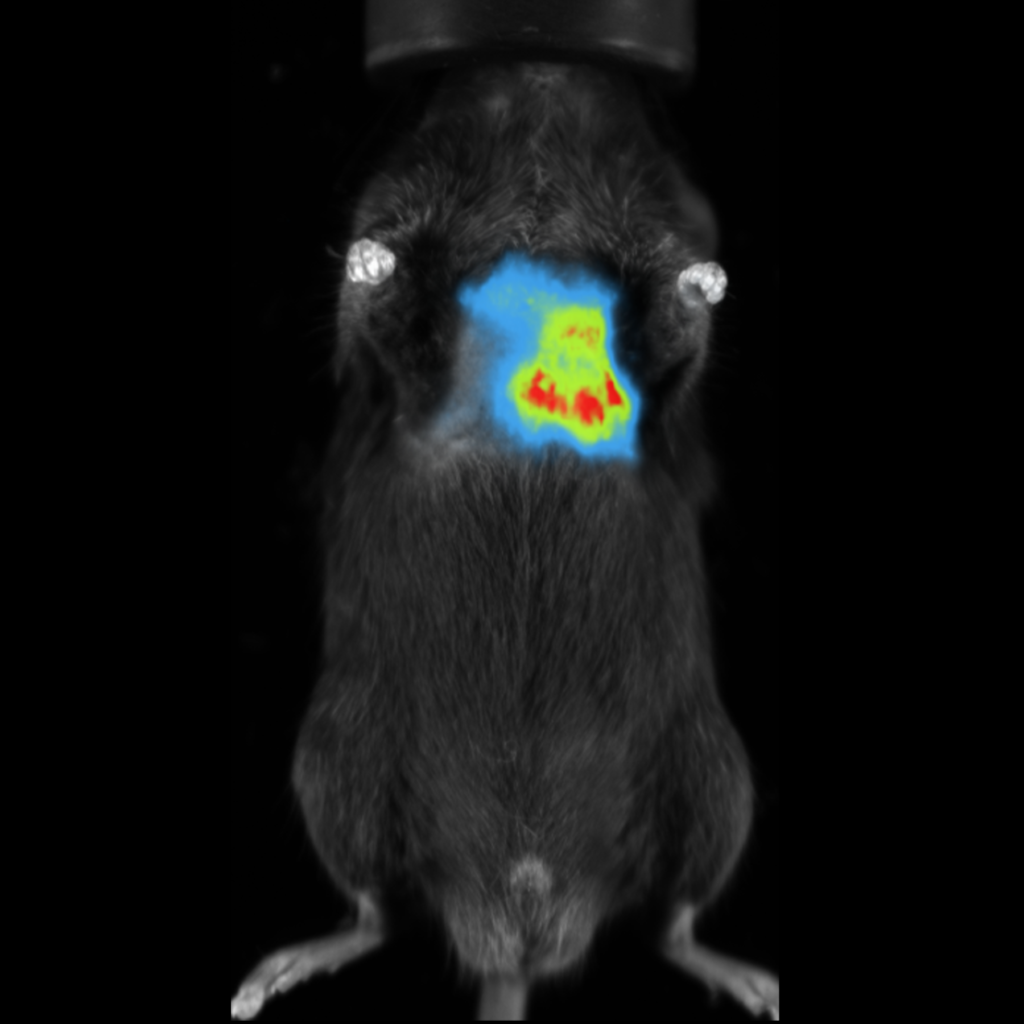
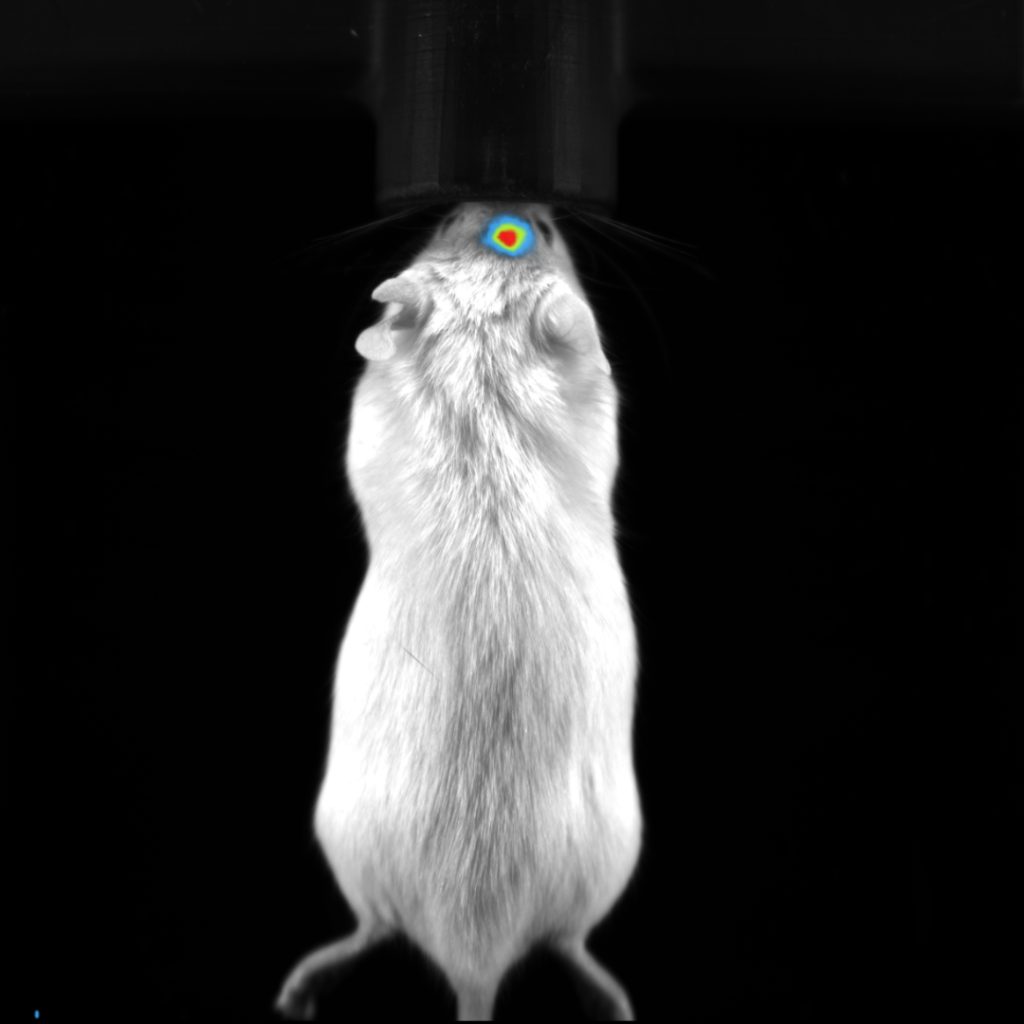
High-Performance Optical Imaging Systems
The Newton 7.0 family of optical imaging systems are manufactured by Vilber, and distributed by Scintica. These systems have been designed specifically for small animal research; they can either image 3 mice (1 rat) or 5 mice (3 rats) to allow for high throughput imaging. The Newton 7.0 has an aperature of f/0.7, grade 0 16-bit scientific CCD camera with 4.6MP resolution. Combined these technical features result in one of the most sensitive preclinical optical imaging systems on the market, while the system includes a heated animal platform with integrated anesthesia and active gas exhaust. The Class 2 lasers ensure even and consistent excitation for fluorescence imaging, while the narrow bandpass emission filters ensure separation of wavelengths for multi-spectral imaging.
The complement of excitation and emission wavelengths on the standard systems allow for a wide range of fluorescent dyes to be imaged, from CFP, GFP, tdTomato, MCherry, Cy5, AlexaFluor 680, DyLight 750, to Nirawave Rocker, etc. While the expanded capabilities into the NIRII range are forthcoming with an updated system with capabilities out to 2000nm, allowing for additional fluorophores to be used.
Each system includes the ability to do 3D BLI tomographic imaging on a single animal. This acquisition protocol results in a 3D representation of where the BLI signal originated, overlayed on a bone and organ atlas which has been adapted to reflect the size and position of the imaging subject.
The analysis software is license free, meaning that as many users as would like can have it installed on their computers to perform analysis on the acquired images at their leisure, without taking up time on the acquisition system.
Overall, the Newton 7.0 is also priced competitively to allow most researchers direct access to the system within their own lab, without the need to access a core imaging lab system which may come with its own challenges such as user fees, difficult scheduling hours, and the need to transport animals
Basic Principles of Optical Imaging
Although optical imaging is quite popular for preclinical in vivo studies, there are some fundamental limitations that are inherent in the imaging modality due to the propagation of light through tissue. The complex nature of living tissue results in many very small structures and boundaries within cells and larger tissue structures, causing the light to scatter greatly and be absorbed specifically in the visible range. Tissue is strongly absorbent of light having short wavelengths, light in the near infrared (NIR) and beyond can penetrate much deeper. However, fluorescent molecules designed in the NIR range are less efficient resulting in less light intensity as those with shorter wavelengths. This makes selecting the most appropriate fluorochrome a very important consideration in one’s study design.
Very specifically, the term fluorescence refers to the emission of a photon caused by a molecule’s transition from an excited electronic state to its ground state. The excited state is achieved by absorption of a photon with sufficient and specific energy, to match that of the difference between the ground and excited state. The wavelength of the emitted photon, as the molecule transitions from excited back to ground state will also have a specific wavelength.
Bioluminescence is a specific form of chemiluminescence in which a photon is emitted when a bioluminescent enzyme metabolizes its specific substrate. The most common example of this is the luciferase-luciferin interaction. The benefits here are that there is no excitation light required, and there is also no background or autofluorescence in this situation. The caveat here is that in order for the photons to be released the substrate, which is often injected, must reach the enzyme, and surrounding conditions must meet all of the requirements for the enzymatic reaction to take place.
In essence, any optical imaging system has three main components – a light source, filters to remove background signal, and a photon detector. When we think about light sources, it is most intuitive to think about fluorescence imaging, as bioluminescence the light is generated during an enzymatic reaction – this will be discussed later. In fluorescence imaging, light sources may be high pressure arc lamps, light emitting diodes (LEDs), or lasers. Most commonly used are LEDs and lasers in small animal imaging.
Filters are used to help improve the signal-to-noise ratio. This is done by filtering out the excitation wavelength, to allow the emission wavelength to be detected alone. There are a variety of filter types which may be used, including long and short pass filters, bandpass filters, and band-rejection filters.
Photon detectors used in preclinical imaging systems are charge-coupled devices (CCDs) or CCD cameras. They are specifically designed for high sensitivity, even in the NIR range, with up to 95% quantum efficiency. These cameras should be cooled to increase sensitivity, as this will reduce dark noise.
In some situations, tomographic imaging may be desirable, to provide some level of information about the depth of the light source, whether it is fluorescence or bioluminescence. For fluorescence tomography, typically several different source positions are chosen. The resulting emission will differ, providing additional information about the interaction of the fluorophore with the excitation source and the travel path of the emitted photon. In bioluminescence tomography there is no excitation source, so spectral imaging must be used. That is observations are made at specific wavelengths, and with this spectrum available, information about the depth of the bioluminescence source can be calculated.
Our Next Modules
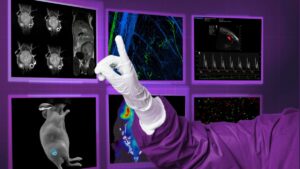
Available Now
Preclinical Imaging Modalities
As we begin to explore the idea of multimodal imaging, let’s first start to explore the variety of preclinical imaging modalities that are most commonly used by researchers around the world.
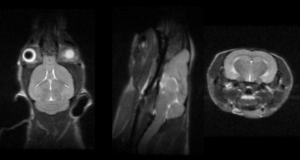
Available Now
Modality: MRI
MRI is considered the gold standard in soft tissue imaging, both in the clinic on patients and by researchers on a wide variety of preclinical imaging subjects.
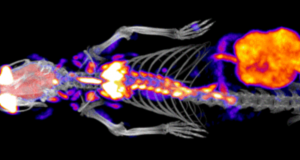
Available Now
Modality: CT
Computed Tomography (CT) is one of the most commonly used clinical imaging techniques, next to perhaps ultrasound.
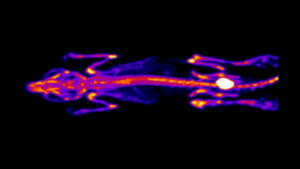
Available Now
Modality: PET
Nuclear imaging modalities include positron emission tomography (PET) as well as single photon emission computed tomography (SPECT), which both detect gamma radiation emitted from a radionucleotide injected into an imaging subject.
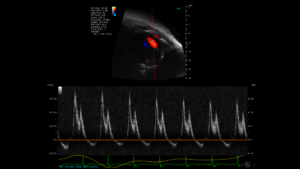
Available Now
Modality: Ultrasound
Ultrasound (US) imaging is one of the most commonly used diagnostic techniques clinically, and is widely used in preclinical imaging as well. It is a safe, non-invasive, and relatively inexpensive technology compared to some of the other available imaging modalities.
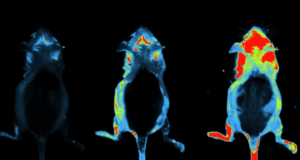
Available Now
Modality: Optical
When discussing Optical Imaging techniques in this series, the meaning is to cover bioluminescence (BLI) and fluorescence (FLI) imaging. T
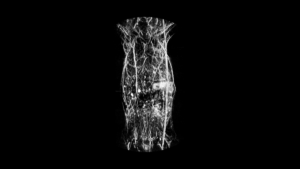
Available Soon
Modality: Photoacoustic
Photoacoustic (PAI) imaging is a technology which combines the sensitivity of optical imaging with the depth of penetration and resolution of ultrasound imaging.
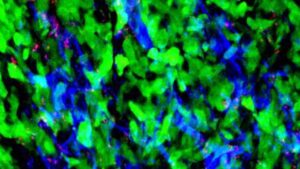
Available Soon
Modality: Microscopy
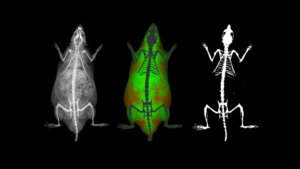