Dual Energy X-Ray Absorptiometry (DXA or DEXA) versus echo magnetic resonance imaging (echo MR):
System Used:
iNSiGHT
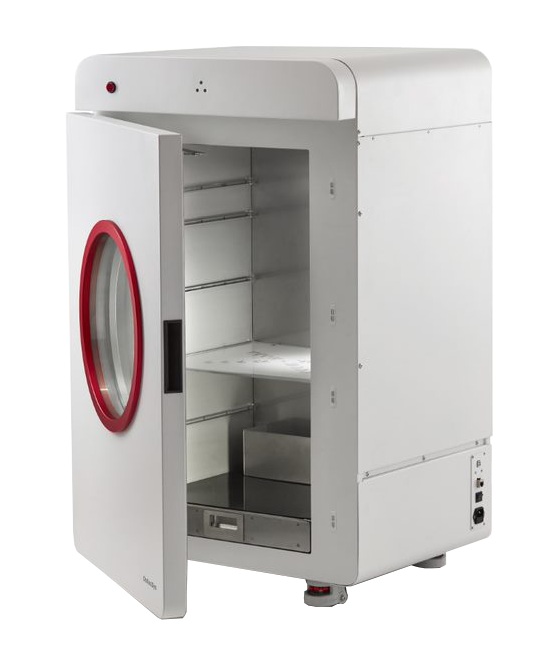
A short case study to compare body composition data collected using these instrumentation
Abstract
Dual Energy X-Ray Absorptiometry (DXA or DEXA) and echo magnetic resonance imaging (echo MR) can assess parameters of body composition such as lean versus fat mass. However, echo MR has the added ability of assessing water content, while DXA has the added ability to assess bone mineral content (BMC) and bone mineral density (BMD), but not water content. Those are the major fundamental difference in terms of parameters collected with these instruments. The purpose of this review is to highlight several recent publications that use both DXA and echo MR and to understand the rationale of using either for the specific data that is collected. The studies ranged from the assessment of cancer-associated cachexia (CAC), the human genetic disease: Down syndrome (DS), and the assessment of activity levels in relation to molecular mechanism involved in changes with body composition. They all had the common factor that the data collected by DXA very closely matched that collected by echo MR. In each case water content was not a parameter measured. This highlights that DXA can be used as a sole instrument in measuring fat versus lean mass if water content is not a consideration, as well as providing the added benefit of measuring BMD and BMC.
Introduction
Dual Energy X-Ray Absorptiometry (DXA or DEXA) and echo magnetic resonance imaging (echo MR) can assess parameters of body composition such as lean versus fat mass. Echo MR is also fundamentally known as nuclear magnetic resonance (NMR); however, this term typically is associated with the chemistry field of research and development and echo MR is better associated with the preclinical and clinical research field. There are fundamental differences between DXA and echo MR that allow these techniques to detect different parameters in terms of body composition, anatomy, and differences seen within a variety of diseases. DXA utilizes an x-ray source which uses both low and high energy beams that will attenuate very differently depending on the tissue these x-rays pass through. The resulting signal intensities are used to determine the amount of bone and soft tissue, by assessing the mass attenuation coefficients at the various x-ray energies. The result of this analysis is an output showing the pixels found to be associated with bone, and the others as soft tissue which is further differentiated into lean and fat mass. This allows DXA to detect mass differences between fat and lean mass and measure the bone mineral content (BMC) and bone mineral density (BMD). BMC is calculated by multiplying the bone density by the bone area.
Echo MR works under the premise of NMR such that every nucleus is electrically charged. When an external magnetic field along with an electromagnetic radiation of a specific frequency is applied there is a resonance transition between magnetic energy levels of an atomic nuclei. This allows the detection of the absorption signals of various atomic nuclei, and this can allow the user to study the structures of molecules quantitatively. In a more clinical or preclinical setting we specifically detect the change in the direction of the rotational axis of protons that are found in water that makes up living tissues. The strong magnetic field forces the protons to align with that field. Then a specified radiofrequency pulse is applied pushing these protons out of equilibrium. When this radiofrequency pulse is then turned off the sensors in the echo MR instrument are then able to detect the energy released from the protons as they realign with the magnetic field. The time it takes for the protons to realign with the magnetic field depends on the chemical nature or environment these protons are found in. This is how an echo MR image can identify different tissue types2. Echo MR deploys this principle to measure masses of fat, lean tissues, and water content. This is also referred to as quantitative magnetic resonance (QMR).
Echo MR and DXA work on two completely different fundamental principles. The major difference is that echo MR can assess water content and DXA is able to measure BMC and BMD. The focus of this review is to highlight a variety of studies that use DXA and echo MR and understand the overlap of the two technologies and when to select one over the other given specific study parameters.

Figure 1. Depiction of how DXA works in terms of using 2 different level of energy x-ray sources to differentiate between soft tissue and bone.

Figure 2. The basic principles of echo MRI. First the protons align with a magnetic field, then are pushed out of line with a radiofrequency pulse, once this pulse is removed, they relax and release a radio signal to be measured.

Table 1. A comparative table highlighting the main differences between DXA and echo MR.
Discussion: DXA and echo MR used in research
Study 1
Most recently Lana-Elola and co-workers published work that highlights the broad range of phenotypic traits in mouse strain for Down Syndrome. Down Syndrome arises due to an extra copy of human chromosome 21 (Hsa21) genes and causes several phenotypic changes; in this study the group used the Dp1Tyb mouse model. This model has an extra copy of 63% of Hsa21-orthologous mouse genes. They were able to identify 468 phenotypic alterations in the mouse model through a series of physiological testing. Among this testing DXA (PIXImus, GE) and echo MR (Bruker) were used as a measurement of phenotypic alterations. Body weight was significantly less in Dp1Tyb mice compared to WT mice from 4 to 16 weeks. Interestingly this trend was only seen between the 4 to 16 weeks within the male mouse model3. This could be due to difference in hormone levels between these models.
This group found that the Dp1Tyb mice have a lower BMD and BMC, however, no difference in lean versus fat mass was observed. In addition to DXA body composition measurements, this study also used the basic x-ray function to identify that Dp1Tyb mice have shorter tibia and skulls. It also identified abnormalities in the shape of the head, snouts, and lips. Due to the altered bone growth in Dp1Tyb mice, they also have skeletal dysmorphology3. The lean versus fat mass was also assessed by echo MR (Echo Medical Systems) and matches the data perfectly collected by the DXA.
This group showed the accuracy of the DXA measurements of body composition, as confirmed using echo MR; of note, however, is that the DXA system also provided measures of BMD and BMC which were found to be altered in this transgenic mouse model of Downs Syndrome. The phenotypic findings in this preclinical model closely match those of the clinical presentation, that is patients are typically shorter in stature, have reduced BMD and BMC, and alterations in their craniofacial morphology4. In addition to assessing the body composition in this model using DXA and echo MR, additional studies were completed to fully characterize the Dp1Tyb mouse model of Down Syndrome. Please refer to the paper for additional details.
Study 2
More recently, in 2021, Luan and co-workers studied the effect cancer-associated cachexia (CAC) has on muscle and adipose tissue in a novel mouse model. CAC is a metabolic effect in response to treatment in advanced cancer patients. It greatly impairs physical functions and accounts for 25% of cancer related deaths12. Luan and coworkers established a novel mouse model that had progressive CAC as tumour growth progressed. They analyzed several biomarkers in the serum of this animal model that included activin A, GDF15, IL-6, IL-1β, and TNF-α. Activin A and GDF15 increased and were correlated with bodyweight decline in this novel mouse model over time. There was a decrease in the cross-sectional area of muscle fibres, and this was correlated to the activation of muscle wasting mechanisms associated with the increase activity of several pathways such as mitogen-activated protein kinases (MAPK). Thermogenesis contributed to adipose tissue wasting as well. Overall, this model would be an excellent candidate as a preclinical aid in better elucidating the molecular mechanism involved with CAC and this can lead to study various interventions 12.
DXA (PIXImus I, GE Lunar) measurements were taken on postnatal day (PD) 64 and PD82 to assess body composition in this novel mouse model of CAC. Regions of interest (ROIs) were drawn to assess the chest, abdomen, and pelvis areas. BMC, bone area, tissue area, ratio of soft-tissue attenuation, total tissue mass, and per cent adiposity (% fat) were measured in the designated ROIs. Echo MR was also used to confirm the lean versus fat body mass. Both DXA and echo MR clearly identified and average of 3.4% decrease in fat mass in the CAC mouse model compared to the control on PD83. Fat mass/body weight also decreased by 62% in the CAC model. When carefully analyzing the DXA data determined the fat (%) to be a mean of 9.8% in the CAC model and 13.2% in the control model. The echo MR data was presented as a ratio of fat mass to body weight, and this directly correlates to the fat (%) calculated by DXA. The data with both DXA and echo MR are very similar indicating the accuracy of using DXA to measure fat and lean mass. This was confirmed by looking at the gross morphology of white adipose tissue and brown adipose tissue. Both white fat and brown fat in the CAC model was decreased on PD65 and PD83. Fat sources involved in energy storage (gonadal fat pad and visceral fat) were also greatly reduced in the CAC model. This reduction in fat within the CAC model was also confirmed by histological staining. All this data confirmed that there is atrophy of adipose tissue due to CAC in this novel mouse model. Once again DXA and echo MR played an integral role in measuring fat tissue in a specific disease model in comparison to a healthy model. This group also measure BMD and BMC in both diseased and healthy animals and saw no significant difference in their specific CAC model. As with the first study examined here, this study confirmed the accuracy of DXA measurements in examining body composition, as compared to measurements made using echo MR.
Conclusion
The above 2 articles use both DXA and echo MR in different research areas. The first focussed on examining a variety of phenotypic traits in a mouse model for Down Syndrome; while the last examined the effects of cancer-associated cachexia. Study 2 examined CAC and the effects on fat deprivation. These studies utilized a variety of experimental techniques, however, this white paper focused on the use of DXA and echo MR to study body composition and bone mineral density/composition. When similar measurements, such as fat and lean mass, were measured using both techniques the results were the same – confirming the accuracy of DXA for such measures. Of note, however, DXA was also able to provide measures of bone mineral density and bone mineral content, which are not possible with echo MR. In both studies, water content, which is a unique measure to echo MRI, was not assessed as a key measure in these disease models.
Recent advances in DXA technology, including the iNSiGHT system from Osteosys, have overcome some of the limitations seen with the PIXImus system used in both studies. Improvements include an increased resolution on the iNSiGHT system (30-100 µm), and scan times improved from 5 minutes on the PIXImus to 25 seconds on the iNSiGHT. Additionally, the iNSiGHT system is self-shielded making it much easier to use within a busy lab space without the need for user protection. The scan area has also been increased on the iNSiGHT, allowing for animals from 10-5000g to be analyzed.
References
1. Ann Laskey, M. & Phil, D. Dual-Energy X-Ray Absorptiometry and Body Composition. Nutrition 12, 45–51 (1996).
2. Kovner, I., Taicher, G. Z. & Mitchell, A. D. Calibration and validation of EchoMRITM whole body composition analysis based on chemical analysis of piglets, in comparison with the same for DXA. http://www.cdc.gov/growthcharts/clinical_charts.htm], (2010).
3. Lana-Elola, E. et al. Comprehensive phenotypic analysis of the dp1tyb mouse strain reveals a broad range of down syndrome-related phenotypes. DMM Disease Models and Mechanisms 14, (2021).
4. LaCombe, J. M. & Roper, R. J. Skeletal dynamics of Down syndrome: A developing perspective. Bone vol. 133 (2020).
5. Granados, J. Z. et al. Activated whole-body arginine pathway in high-active mice. PLoS ONE 15, (2020).
6. Timothy Lightfoot, J. et al. Strain screen and haplotype association mapping of wheel running in inbred mouse strains. J Appl Physiol 109, 623–634 (2010).
7. Serra-Prat, M. et al. Intracellular water content in lean mass is associated with muscle strength, functional capacity, and frailty in community-dwelling elderly individuals. A cross-sectional study. Nutrients 11, (2019).
8. Roumelioti, M.-E. et al. Fluid balance concepts in medicine: Principles and practice. World Journal of Nephrology 7, 1–28 (2018).
9. Tamma, G. et al. Aquaporin membrane channels in oxidative stress, cell signaling, and aging: Recent advances and research trends. Oxidative Medicine and Cellular Longevity vol. 2018 (2018).
10. Cowen, L. E., Hodak, S. P. & Verbalis, J. G. Age-Associated Abnormalities of Water Homeostasis. Endocrinology and Metabolism Clinics of North America 42, 349–370 (2013).
11. Puig-Domingo, M. et al. Muscle strength in the Mataró aging study participants and its relationship to successful aging Aging Clinical and Experimental Research. Aging Clin Exp Res vol. 20 (2008).
12. Luan, Y. et al. Development of ovarian tumour causes significant loss of muscle and adipose tissue: a novel mouse model for cancer cachexia study. Journal of Cachexia, Sarcopenia and Muscle (2022) doi:10.1002/jcsm.12864.
13. Zwickl, H. et al. Effect of cachexia on bone turnover in cancer patients: a case-control study. BMC Cancer 21, (2021).