With the ever-growing preclinical imaging field, it is difficult to know which imaging modality is ideal for your experiment. This brief overview aims to help researchers optimize their experiments and their funding by choosing ideal imaging modalities for their prospective publications. Modalities discussed include:
- Magnetic Resonance Imaging (MRI)
- Positron Emission Tomography (PET)
- Computed Tomography (CT)
- Ultrasound (US)
- Optical in vivo Bioluminescence/Fluorescence Imaging
By explaining the functionality of each imaging modality in research, this article will allow researchers to feel confident in their experiments and hopefully minimize negative reviewer feedback. Combinations of imaging modes are also touched upon.
Magnetic Resonance Imaging

Magnetic resonance imaging (MRI) is a technique that utilizes magnetic fields to impact proton alignment within tissue, generating images of the anatomy and physiological processes within the body. Specifically, MRI, the gold standard in soft tissue imaging, offers researchers the ability to distinctly discern normal and pathological tissue. Further, multi-modal imaging, allows researchers to gather more relevant information about the soft tissue and its surrounding microenvironment. Examples include PET/MRI, or even 3D optical images co-registered with a whole-body MRI image.
Ideally, MRI is used for looking at soft tissue anatomy and malignancies. Combining MRI with other technologies, like PET, allows for researchers to customize their images for the disease model of interest.
Until the recent availability of affordable, easy to use, and compact MR systems, such as the M-Series from Aspect Imaging, high quality, longitudinal studies have not been as readily accessible in preclinical research. With the availability of these systems, researchers will be able to afford high quality images for their publications that better identify pathological outcomes associated with their disease models. Further, the emergence of the compact imaging field allows novice users to quickly gain experience in imaging modalities that were once left for specialized technicians.
Positron Emission Tomography
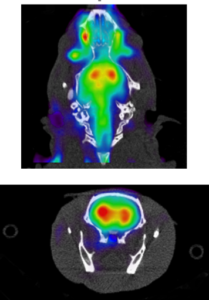
Positron Emission Tomography (PET) provides information on the metabolic or biochemical activity present in a given anatomical region using radiotracers. PET is often combined with an anatomy-based imaging modality allowing researchers to get a complete picture of what is happening inside a specimen’s body. Frequently, PET is combined with computed tomography (CT) because of its associated costs and ease of use; however, MRI is growing in popularity as an image accompanying PET images. PET/MRI is especially common in oncology studies, as the MRI allows for better rendering of soft tissue.
PET imaging requires radiotracers that mark the metabolite or chemical in the body that is being examined. A radiotracer is a chemical compound in which atoms have been replaced by a radionuclide. After undergoing normal radioactive decay, the path the radiotracer takes can be traced, from reactants to products. Essentially, a radiotracer allows for the visualization of chemical processes within the body. Fluorodeoxyglucose (FDG) is the most commonly used radiotracer, although many others exist or are being studied for future use.
PET imaging is ideal for metabolic, neurology, pathology, cardiology, oncology, and toxicology studies. Because of the variety of available chemical compounds to use with PET, there are many research topics that can be addressed with convenient access to PET/MRI or PET/CT system. Unfortunately, because of the price of clinical PET equipment, PET studies have been done infrequently and with little repetition. Fortunately, preclinical PET systems, offered by Sedecal, are an emerging area of laboratory technology that offer high quality, quick, and affordable options for researchers who are hoping to be at the cutting edge of their field.
Computed Tomography

Computed tomography, or CT, imaging combines X-ray images taken of the body at different angles to create cross sectional images of the bones, blood vessels, and internal soft tissues. CT scans allow medical professionals to view parts of the body in individual slices.
CT is frequently used in combination with other imaging modalities, like PET/CT, to answer complex questions about biochemical changes across the body associated with disease models. CT is also a great diagnostic tool that provides better imaging quality and better pre-surgical anatomical rendering than X-ray imaging alone. As seen in the image at the left, CT is great for determining approximate volumes of regions in body such as the skull or tumors.
CT is readily available in a hospital setting but is frequently quite expensive for researchers given the specialized technician and/or core facility they must hire to run their studies. Further, the CT equipment is often large and in a central location to the hospital making it difficult to access. Thankfully, the emergence of compact, preclinical imaging allows researchers to access their personal CT whenever they need to. The trend towards user-friendly imaging equipment, like Sedecal’s PET/CT system, allows novice users to quickly learn the necessary settings and gating to build high quality, comprehensive images.
High Frequency Ultrasound

Ultrasound (US) has become a common preclinical imaging modality that can be used to visualize a variety of internal structures within small animal models with high resolution (down to 30µm). A high frequency sound wave beam is directed toward the tissue of interest, and the reflected waves received by the imaging probe create an image. This provides researchers with primary anatomical information in real-time allowing for the non-invasive identification and quantification of organ/tumor parameters in both two- and three-dimensions, as well as measurements related to cardiac function and blood flow.
Ultrasound is ideal for general anatomical imaging, assessment of cardiovascular system function, tumor imaging, tissue perfusion, US-guided needle injections, drug or gene delivery via sonoporation, and shear wave elastography.
While US imaging is the most readily available preclinical tool, recent advancements in the field have allowed for compact, preclinical devices that are easily taught to novices as well as fully customizable to more advance users. For example, the S-Sharp Prospect T1 provides small animal researchers the ability to visualize and quantify anatomical targets, hemodynamics and therapeutic interventions with a resolution down to 30µm. This is a cost-effective (3 to 4 times less expensive than comparable systems) system that allows individual preclinical labs to perform ultrasound imaging on their own schedule without the need to try to find availability or pay per-usage fees charged by some imaging cores.
Bioluminescence/Fluorescence Imaging

Perhaps a lesser known imaging modality is bioluminescence and fluorescence imaging (BFI). BFI is ideal for in vivo longitudinal imaging, as well as ex vivo and in vitro applications. When using cell lines expressing a luciferase or fluorescent reporter, or injecting fluorescently tagged molecules, various targets can be visualized and tracked using these systems. This imaging modality is non-invasive, and radiation-free allowing users to image multiple animals at a time. BFI is primarily used in tumor imaging, metastasis tracking, cell migration studies, molecule targeting, and visualization of the vasculature and microcirculation.
In addition to bioluminescence, fluorescence is a useful tool for tracking molecules, cells and structures over time. With several novel fluorescent probes available to researchers, an optical imaging system requires suitable light sources covering the light spectrum and detection filters to accommodate a variety of signals. Comprehensive systems like the Newton 7.0 by Vilber can support up to 8 excitation channels in the infrared, near infrared, visible RGB and UV spectra, where signals can also be overlaid so that several reporters can be visualized simultaneously. Furthermore, the quality of optical hardware is important to ensure high light collection capacity for optimal signal sensitivity. Using highly-sensitive optics, the fluorescence or bioluminescence signal intensity can be quantified to demonstrate changes in cell populations or migration of cells over time, for example. The compact and customizable Newton 7.0 uses proprietary optics and CCD camera capturing hardware to detect bioluminescence and fluorescence signals at the femtogram and picogram level, respectively. Furthermore, it offers scalable experiment design with an imaging platform accommodating up to 5 mice.
Conclusion
Overall, researchers are required to provide comprehensive images to help explain their complex studies to reviewers. In the past, researchers have been forced to use less-quality imaging capabilities due to financial or time constraints. With the emergence of the compact preclinical imaging field, companies like Aspect Imaging, Sedecal, Vilber, and S-Sharp have catered imaging equipment to the preclinical researcher. Now high quality, quick, reliable images are also affordable and will ensure researchers have competitive, high quality data for their publications as well as future grant endeavors.
Should you be interested in more information on any of these imaging modalities, please visit the imaging section at www.scintica.com or contact Brianna Sellers at 1-519-914-5495 ext 20 (info@scintica.com).